Fluorescent cells could be key to cancer diagnosis
Scientists at the University of Adelaide are a step closer to creating a fluorescent sensor that detects cancerous cells, which could become a new tool in the early identification of cancer.
They have also created a new peptide which may prevent the replication of cancerous cells and lead to a new cancer treatment.
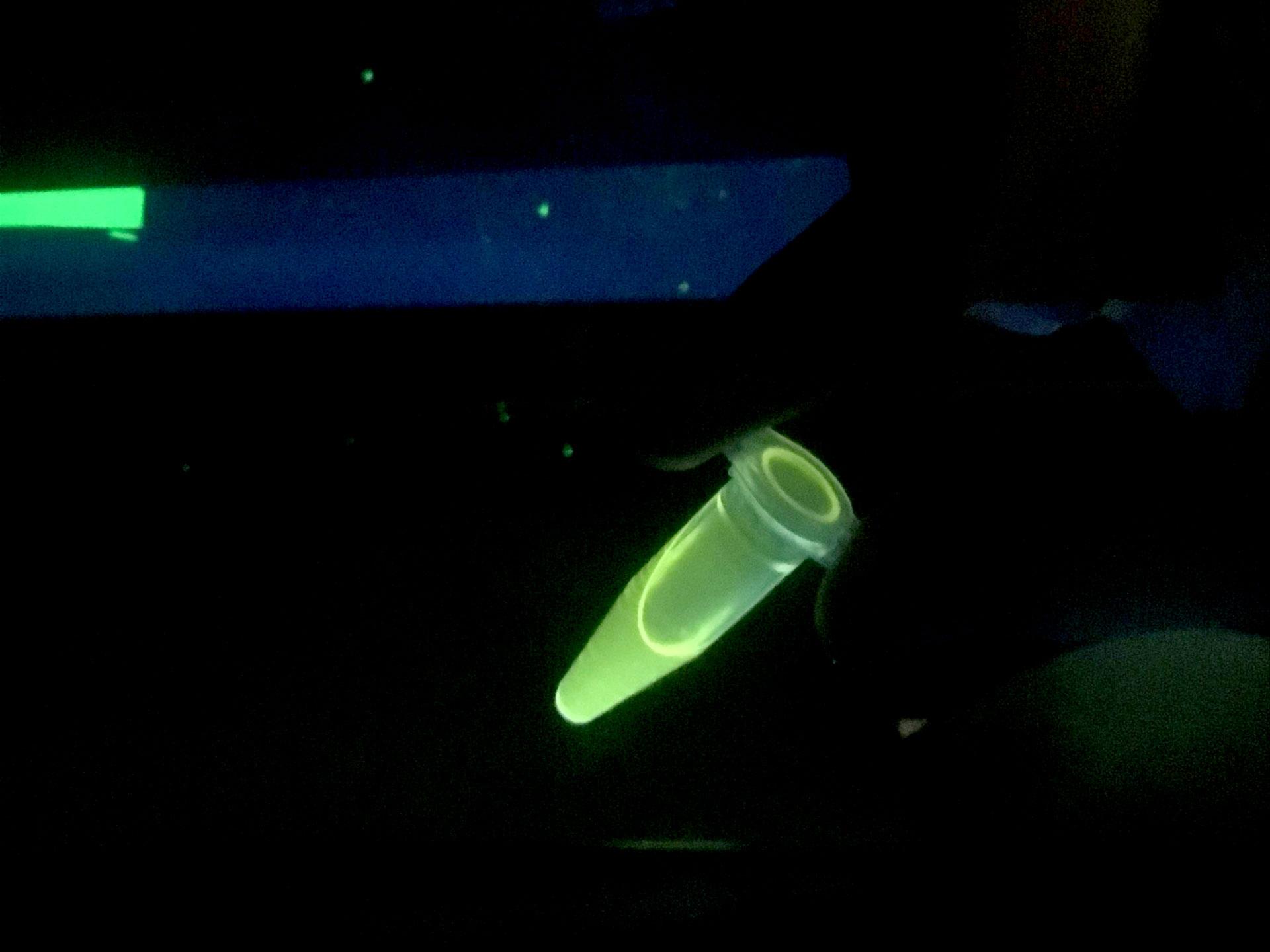
Glow in the dark: The fluorescent peptide glows yellow under UV light. Image by Aimee Horsfall.
Glow in the dark peptides
Collaborative research involving University of Adelaide chemists and biomedical scientists has developed a peptide which could make cancerous cells ‘glow in the dark’ to distinguish them between healthy cells.
“We have developed a peptide that interacts with the protein PCNA, which is present in high levels in cancerous cells, and upon interacting gives out a fluorescent response that we can detect,” says lead author Dr Aimee Horsfall, who completed her PhD recently in the University of Adelaide's School of Physical Sciences.
“This is the first step toward developing a sensor that may be useful in distinguishing between healthy cells and cancerous cells.”Dr Aimee Horsfall
PCNA is a human protein that is essential to DNA-replication and repair. Consequently, it is found in high levels in cancerous cells and therefore inhibiting its function is a potential target for development of new cancer treatments.
The research published in Bioorganic & Medicinal Chemistry Letters details how scientists used a peptide that is known to interact with PCNA, and built into it fluorophores which are environmentally sensitive.
“These fluorophores are not fluorescent in water, like the inside of a cell; but are highly fluorescent in hydrophobic and oil-like environments, like patches on the surface of a protein” said study co-author and MPhil candidate Theresa Chav.
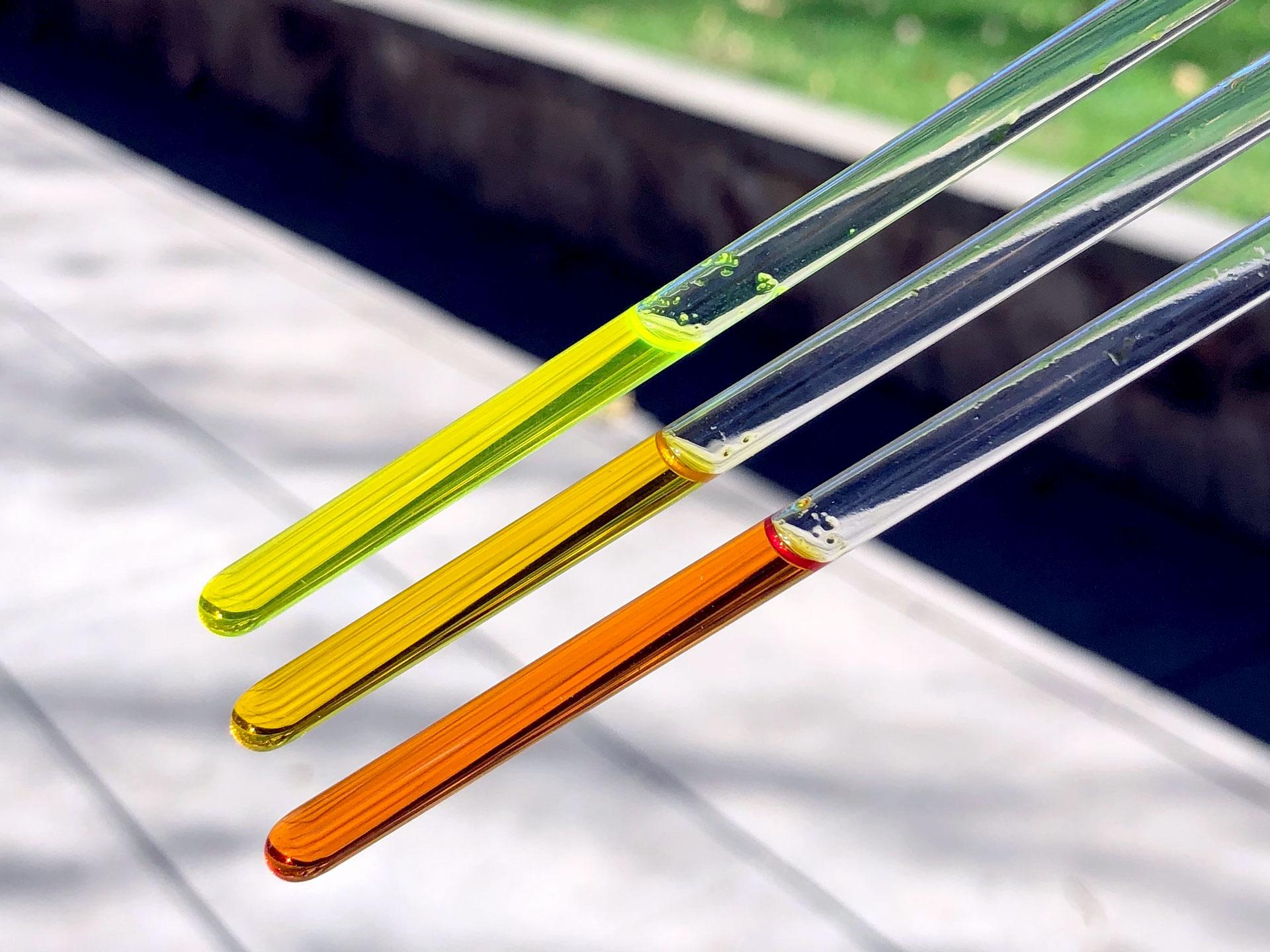
Going for gold: After synthesising a new chemical, chemists separate the desired product from the rest by collecting small fractions and checking their purity. Image by Aimee Horsfall
“Smart design, using a 3D model of our peptide bound to our scaffolding peptide, allowed us to strategically position the fluorophore within the peptide so that when the peptide was in water it was not fluorescent, but on interacting with the protein PCNA the fluorophore became embedded onto the protein surface and gives a large fluorescent output that we can detect. These peptides therefore act as a ‘sensor’ for PCNA,” said Ms Chav.
The researchers compared two different fluorophores, and three different positions within the peptide and were able to show that only one position was suitable to incorporate the fluorophore and maintain an interaction with PCNA; and the fluorescence did increase in the presence of PCNA.
Preventing the replication of cancerous cells
In related research published in the Journal of Biological Chemistry, Dr Horsfall and her chemistry and biochemistry colleagues have designed a new peptide that binds to PCNA with higher affinity than anything else that has been previously reported.
Known as a PCNA-interacting protein-box – or PIP-Box for short – researchers uncovered a few changes that either help ‘stick’ the peptide onto the protein surface better; and that stabilise the peptide structure when it is bound to PCNA.
“This structure is important because, like a jigsaw puzzle in 3D, the shape defines how the two halves of the puzzle fit together,” said University of Adelaide PhD student and study co-author Beth Vandborg.
“Here, we know peptides that interact with PCNA like to take on a single loop which we call a 310-helical turn. Positioning a positively charged, and a negatively charged group on either side of that turn appears to help stabilise that turn, where the two halves are attracted to one another because of the opposing charge like ends of a magnet.”
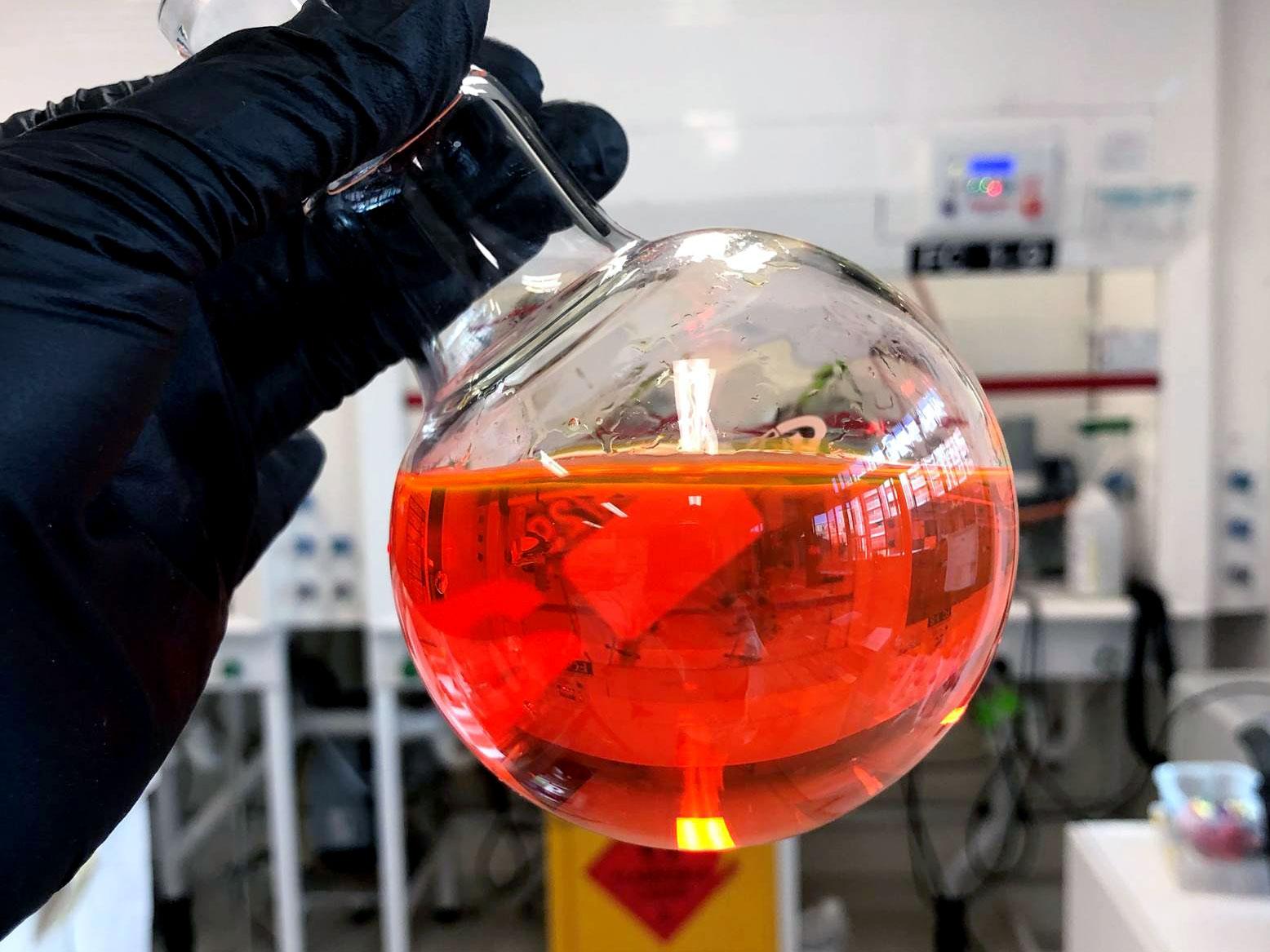
Staring into the crystal ball: An orange reaction mixture en route to making a fluorescent peptide. Image by Aimee Horsfall.
In a related publication in RSC Chemical Biology, the researchers show that by physically linking these two parts of the peptide, they could stabilise this shape before the peptide interacted with PCNA.
Five different types of linkers were trialled, and the best candidate uses a fluorescent linker developed in Dr Horsfall’s Masters of Philosophy project.
“Most linkers we use for this kind of work are not fluorescent, which means to study the resulting peptide in cells we need to subsequently attach a fluorescent molecule so we can see the peptide using fluorescence microscopy. Our linker is special because it is inherently fluorescent, which means we don’t need to modify our peptide any further to be able to test in cells and see where it goes,” said Dr Horsfall.
“We wanted to demonstrate this and tested our fluorescent linked peptide out in breast cancer cells, and were really excited to see blue fluorescence inside the cells indicating our linked peptide managed to get in, whereas an unlinked version couldn’t.”
The discovery provides an important new scaffold from which to develop a potential cancer therapeutic.
“This information can be leveraged to design a molecule that binds PCNA really well. A version of this molecule may be able to inhibit PCNA function, therefore hopefully shutting down DNA-replication,” said Ms Vandborg.
“If we can shut down replication in cancerous cells, we can stop their spread and thereby have created a new cancer treatment.”
“Our next goal is to try and control where the peptide goes inside the cell, to reach a compartment called the nucleus where PCNA is found, so we can then test if our peptide can stop DNA-replication.”
The ongoing research is led by Professor Andrew Abell from the School of Physical Sciences and Dr John Bruning in the School of Biological Sciences.
Sciences research in focus
- A turn-on fluorescent PCNA sensor - Bioorganic & Medicinal Chemistry Letters
- Unlocking the PIP-box: A peptide library reveals interactions that drive high-affinity binding to human PCNA - Journal of Biological Chemistry
- A cell permeable bimane-constrained PCNA-interacting peptide - RSC Chemical Biology